Coxiella burnetii
From Wikipedia the free encyclopedia
Coxiella burnetii | |
---|---|
![]() | |
A dry fracture of a Vero cell exposing the contents of a vacuole where Coxiella burnetii is growing | |
Scientific classification ![]() | |
Domain: | Bacteria |
Phylum: | Pseudomonadota |
Class: | Gammaproteobacteria |
Order: | Legionellales |
Family: | Coxiellaceae |
Genus: | Coxiella |
Species: | C. burnetii |
Binomial name | |
Coxiella burnetii (Derrick 1939) Philip 1948 |
Coxiella burnetii is an obligate intracellular bacterial pathogen, and is the causative agent of Q fever.[1] The genus Coxiella is morphologically similar to Rickettsia, but with a variety of genetic and physiological differences. C. burnetii is a small Gram-negative, coccobacillary bacterium that is highly resistant to environmental stresses such as high temperature, osmotic pressure, and ultraviolet light. These characteristics are attributed to a small cell variant form of the organism that is part of a biphasic developmental cycle, including a more metabolically and replicatively active large cell variant form.[2] It can survive standard disinfectants, and is resistant to many other environmental changes like those presented in the phagolysosome.[3]
History and naming
[edit]Research in the 1920s and 1930s identified what appeared to be a new type of Rickettsia, isolated from ticks, that was able to pass through filters. The first description of what may have been Coxiella burnetii was published in 1930 by Hideyo Noguchi, but since his samples did not survive, it remains unclear as to whether it was the same organism. The definitive descriptions were published in the late 1930s as part of research into the cause of Q fever, by Edward Holbrook Derrick and Macfarlane Burnet in Australia, and Herald Rea Cox and Gordon Davis at the Rocky Mountain Laboratory (RML) in the United States.[4]
The RML team proposed the name Rickettsia diaporica, derived from the Greek word for having the ability to pass through filter pores, to avoid naming it after either Cox or Davis if indeed Noguchi's description had priority. Around the same time, Derrick proposed the name Rickettsia burnetii, in recognition of Burnet's contribution in identifying the organism as a Rickettsia. As it became clear that the species differed significantly from other Rickettsia, it was first elevated to a subgenus named after Cox, Coxiella, and then in 1948 to its own genus of that name, proposed by Cornelius B. Philip, another RML researcher.[4] Research in the 1960s–1970s by French Canadian-American microbiologist and virologist Paul Fiset was instrumental in the development of the first successful Q fever vaccine.[5]
Coxiella was difficult to study because it could not be reproduced outside a host. However, in 2009, scientists reported a technique allowing the bacteria to grow in an axenic culture and suggested the technique may be useful for study of other pathogens.[6]
Pathogenesis
[edit]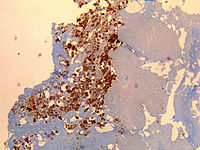
Of the many C. burnetii strains, two of the most studied are the Nine Mile phase I and Priscilla phase I strain. In recent years, more strains have been studied. Nonetheless, it has been demonstrated that the Nine Mile strain is one of the most virulent strains of C. burnetti with as few as four organisms needed to cause infection. This is particularly relevant as murine rodents are poorly susceptible to C. burnetii, necessitating a higher dose and a more virulent dose to inoculate murine rodents for disease study. [7]
The ID50 (the dose needed to infect 50% of experimental subjects) is one via inhalation; i.e., inhalation of one organism will yield disease in 50% of the population. This is an extremely low infectious dose (only 1-10 organisms required), making C. burnetii one of the most infectious known organisms.[8][9] Disease occurs in two stages: an acute stage that presents with headaches, chills, and respiratory symptoms, and an insidious chronic stage.
C. burnettii infections begins within the alveoli. Upon inhalation, it targets alveolar macrophages and passively enters them via actin-dependent phagocytosis. After initial binding, it is suggested that C. burnetii enters phagocytotic cells via passive actin-dependent phagocytosis and enters non-professional phagocytes via an active zipper mechanism. C. burnetii exploits the αVβ3 integrin to enter using RAC1-dependent phagocytosis, which is believed to have evolved as a mechanism to avoid the induction of an inflammatory response.[10]
Following infection, C. burnetii has a biphasic developmental cycle, which consists of small cell variant (SCV) and large cell variant (LCV) morphological forms, which are both infectious. As the SCV is metabolically repressed and resistant to many environmental stressors, it is likely the form that initiates natural infections. Having entered a host cell, C. burnetii SCVs transit through the phagolysosomal maturation pathway. In the first six hours post-infection, endosomes, autophagosomes, and lysosomes containing acid phosphatase fuse with the nascent phagosome to form early PV, which fosters the transition from SCV to LCV. Resultantly, C. burnetii is metabolically activated and produces the T4SS to translocate effector proteins into the host cytoplasm. After 6 days, C. burnetii transitions back to SCV.[7][11]
While most infections clear up spontaneously, treatment with tetracycline or doxycycline appears to reduce the symptomatic duration and reduce the likelihood of chronic infection. A combination of erythromycin and rifampin is highly effective in curing the disease, and vaccination with Q-VAX vaccine (CSL) is effective for prevention of it.[citation needed]
The bacteria use a type IVB secretion system known as Icm/Dot (intracellular multiplication / defect in organelle trafficking genes) to inject over 100 effector proteins into the host. These effectors increase the bacteria's ability to survive and grow inside the host cell by modulating many host cell pathways, including blocking cell death, inhibiting immune reactions, and altering vesicle trafficking.[12][13][14] In Legionella pneumophila, which uses the same secretion system and also injects effectors, survival is enhanced because these proteins interfere with fusion of the bacteria-containing vacuole with the host's degradation endosomes.[15]
Use as a biological weapon
[edit]The United States ended its biological warfare program in 1969. When it did, C. burnetii was one of seven agents it had standardized as biological weapons.[16]
Genomics
[edit]At least 75[17] completely sequenced genomes of Coxiella burnetii strains exist,[18] which contain about 2.1 Mbp of DNA each and encode around 2,100 open reading frames; 746 (or about 35%) of these genes have no known function.
In bacteria small regulatory RNAs are activated during stress and virulence conditions. Coxiella burnetii small RNAs (CbSRs 1, 11, 12, and 14) are encoded within intergenic region (IGR). CbSRs 2, 3, 4 and 9 are located antisense to identified ORFs. The CbSRs are up-regulated during intracellular growth in host cells.[19]
All C. burnetii isolates either carry one of four conserved independently-replicating large plasmids (QpH1, QpDG, QpRS, or QpDV) or a chromosomal element derived from QpRS. QpH1 carries virluence factors important for the bacterium's survival inside mouse macrophages[20] and Vero cells; growth on axenic media is unaffected. QpH1 also contains a toxin-antitoxin system.[21] Among all plasmids, 8 conserved genes code for proteins that are inserted into the host cell via the secretion system.[21]
Additional images
[edit]- C. burnetii, the causative agent of Q fever
References
[edit]- ^ Shaw EI, Voth DE (January 2019). "Coxiella burnetii: A Pathogenic Intracellular Acidophile". Microbiology. 165 (1): 1–3. doi:10.1099/mic.0.000707. PMC 6600347. PMID 30422108.
- ^ Voth DE, Heinzen RA (April 2007). "Lounging in a lysosome: the intracellular lifestyle of Coxiella burnetii". Cellular Microbiology. 9 (4): 829–40. doi:10.1111/j.1462-5822.2007.00901.x. PMID 17381428.
- ^ Sankaran N (2000). "Coxiella burnetii". Microbes and people : an A-Z of microorganisms in our lives. Phoenix, Arizona: The Oryx Press. pp. 72. ISBN 1-57356-217-3. "In contrast to other rickettsiae, which are highly sensitive and easily killed by chemical disinfectants and changes in their surroundings, C. burnetii is highly resistant" & "Q fever". Centers for Disease Control and Prevention; National Center for Infectious Diseases; Division of Viral and Rickettsial Diseases; Viral and Rickettsial Zoonoses Branch. 2003-02-13. Retrieved 2006-05-24. "The organisms are resistant to heat, drying, and many common disinfectants."
- ^ a b McDade JE (1990). "Historical Aspects of Q Fever". In Marrie TJ (ed.). Q Fever, Volume I: The Disease. CRC Press. pp. 5–22. ISBN 0-8493-5984-8.
- ^ Saxon, Wolfgang (March 8, 2001). "Dr. Paul Fiset, 78, Microbiologist And Developer of Q Fever Vaccine". New York Times. p. C-17.
- ^ Omsland A, Cockrell DC, Howe D, Fischer ER, Virtaneva K, Sturdevant DE, et al. (March 2009). "Host cell-free growth of the Q fever bacterium Coxiella burnetii". Proceedings of the National Academy of Sciences of the United States of America. 106 (11): 4430–4. Bibcode:2009PNAS..106.4430O. doi:10.1073/pnas.0812074106. PMC 2657411. PMID 19246385.
- ^ a b Eldin, Carole; Mélenotte, Cléa; Mediannikov, Oleg; Ghigo, Eric; Million, Matthieu; Edouard, Sophie; Mege, Jean-Louis; Maurin, Max; Raoult, Didier (January 2017). "From Q Fever to Coxiella burnetii Infection: a Paradigm Change". Clinical Microbiology Reviews. 30 (1): 115–190. doi:10.1128/CMR.00045-16. ISSN 0893-8512. PMC 5217791. PMID 27856520.
- ^ Tigertt WD, Benenson AS, Gochenour WS (September 1961). "Airborne Q fever". Bacteriological Reviews. 25 (3): 285–93. doi:10.1128/br.25.3.285-293.1961. PMC 441106. PMID 13921201.
- ^ "Q fever caused by Coxiella burnetii". Centers for Disease Control. 15 January 2019.
- ^ Dragan, Amanda L.; Voth, Daniel E. (April 2020). "Coxiella burnetii: international pathogen of mystery". Microbes and Infection. 22 (3): 100–110. doi:10.1016/j.micinf.2019.09.001. ISSN 1286-4579. PMC 7101257. PMID 31574310.
- ^ Dragan, Amanda L.; Voth, Daniel E. (2020-04-01). "Coxiella burnetii: international pathogen of mystery". Microbes and Infection. 22 (3): 100–110. doi:10.1016/j.micinf.2019.09.001. ISSN 1286-4579. PMC 7101257. PMID 31574310.
- ^ Lührmann A, Nogueira CV, Carey KL, Roy CR (November 2010). "Inhibition of pathogen-induced apoptosis by a Coxiella burnetii type IV effector protein". Proceedings of the National Academy of Sciences of the United States of America. 107 (44): 18997–9001. Bibcode:2010PNAS..10718997L. doi:10.1073/pnas.1004380107. PMC 2973885. PMID 20944063.
- ^ Clemente TM, Mulye M, Justis AV, Nallandhighal S, Tran TM, Gilk SD (October 2018). Freitag NE (ed.). "Coxiella burnetii Blocks Intracellular Interleukin-17 Signaling in Macrophages". Infection and Immunity. 86 (10). doi:10.1128/IAI.00532-18. PMC 6204741. PMID 30061378.
- ^ Newton HJ, Kohler LJ, McDonough JA, Temoche-Diaz M, Crabill E, Hartland EL, Roy CR (July 2014). Valdivia RH (ed.). "A screen of Coxiella burnetii mutants reveals important roles for Dot/Icm effectors and host autophagy in vacuole biogenesis". PLOS Pathogens. 10 (7): e1004286. doi:10.1371/journal.ppat.1004286. PMC 4117601. PMID 25080348.
- ^ Pan X, Lührmann A, Satoh A, Laskowski-Arce MA, Roy CR (June 2008). "Ankyrin repeat proteins comprise a diverse family of bacterial type IV effectors". Science. 320 (5883): 1651–4. Bibcode:2008Sci...320.1651P. doi:10.1126/science.1158160. PMC 2514061. PMID 18566289.
- ^ Croddy, Eric C.; Hart, C. Perez-Armendariz J. (2002). Chemical and Biological Warfare. Springer. pp. 30–31. ISBN 0-387-95076-1.
- ^ Abou Abdallah, Rita; Million, Matthieu; Delerce, Jeremy; Anani, Hussein; Diop, Awa; Caputo, Aurelia; Zgheib, Rita; Rousset, Elodie; Sidi Boumedine, Karim; Raoult, Didier; Fournier, Pierre-Edouard (21 November 2022). "Pangenomic analysis of Coxiella burnetii unveils new traits in genome architecture". Frontiers in Microbiology. 13. doi:10.3389/fmicb.2022.1022356. PMC 9721466. PMID 36478861.
- ^ "Genome - NCBI". National Center for Biotechnology Information, U.S. National Library of Medicine. Archived from the original on 2011-11-28. Retrieved 1 January 2022.
- ^ Warrier I, Hicks LD, Battisti JM, Raghavan R, Minnick MF (2014). "Identification of novel small RNAs and characterization of the 6S RNA of Coxiella burnetii". PLOS ONE. 9 (6): e100147. Bibcode:2014PLoSO...9j0147W. doi:10.1371/journal.pone.0100147. PMC 4064990. PMID 24949863.
- ^ Luo, Shengdong; Lu, Shanshan; Fan, Huahao; Chen, Zeliang; Sun, Zhihui; Hu, Yan; Li, Ruisheng; An, Xiaoping; Uversky, Vladimir N.; Tong, Yigang; Song, Lihua (8 April 2021). "The Coxiella burnetii QpH1 Plasmid Is a Virulence Factor for Colonizing Bone Marrow-Derived Murine Macrophages". Journal of Bacteriology. 203 (9). doi:10.1128/jb.00588-20. PMC 8092169. PMID 33558394.
- ^ a b Wachter, S; Cockrell, DC; Miller, HE; Virtaneva, K; Kanakabandi, K; Darwitz, B; Heinzen, RA; Beare, PA (December 2022). "The endogenous Coxiella burnetii plasmid encodes a functional toxin-antitoxin system". Molecular Microbiology. 118 (6): 744–764. doi:10.1111/mmi.15001. PMC 10098735. PMID 36385554.
External links
[edit]
- Coxiella burnetii genomes and related information at PATRIC, a Bioinformatics Resource Center funded by NIAID